|
 |
10. Separation
An important observation is that fluid flow is always irreversible. It is
irreversible because fluids have viscosity, and whenever velocity gradients appear in the
flow there will be friction and energy dissipation due to viscous stresses. The flow
can be reversible only if there are no velocity gradients anywhere, since that is the
only condition under which there is no friction. However, when frictional effects are
small, a flow may be approximately reversible. For instance, in the flow
through a large duct where the boundary layers are very thin, viscous effects are confined
to a rather small region, and the fluid friction may sometimes be neglected. This is not
the case for most practical flows. In most pipe and duct flows, for example, the velocity
gradients extend over the entire cross-section and frictional stresses are all important.
Even if the boundary layers are thin to begin with, they can thicken rapidly under some
circumstances, and the flow can separate, as in the diffuser flow shown in
figure 10.1.
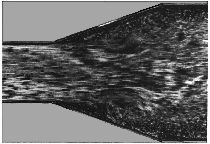 |
 |
Figure 10.1 Separated diffuser flow (included angle =20^o). Flow is from left to
right. From Japan Society of Mechanical Engineers.
|
If the flow is in the direction of
increasing area (this is called a diffuser flow), the effects of friction and separation
are usually small, if the angle of divergence is very small. For larger
angles (an included angle
greater than about 7^o is sufficient), there is a possibility of
flow separation, by which we mean that in some parts of the flow the fluid is actually
going in a direction opposite to the bulk flow direction. Large losses can occur, as the
mechanical energy of the fluid is used to drive large unsteady eddying motions which
eventually dissipate into heat. This type of flow can occur for quite small angles. In
contrast, when the flow is in the direction of decreasing area,
as in the contraction flow shown in figure 10.2, there is very little risk of
producing large separated regions, even for very large values of
the included angle (up to 45^o), and generally losses are quite small.
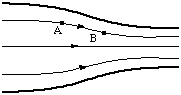 |
 |
Figure 10.2 Flow in a contraction. Flow is from left to right.
|
So great care needs to be taken in
considering frictional effects: sometimes they are negligible, and sometimes they are not.
When separation occurs, however, frictional effects are always important. It is worth
noting that sharp corners almost always produce a separated flow. The sudden expansion and
contraction flows shown in figure 10.3
graphically demonstrate the problem, and these flows are clearly irreversible.
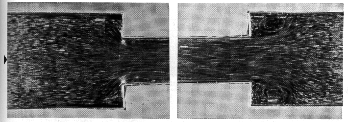 |
 |
Figure 10.3 Flow through a sudden contraction (left), and a sudden expansion
(right). Flow is from left to right. From Japan Society of Mechanical Engineers.
|
Sharp corners typically produce a separated flow, but they are not the only cause of
separation. For example, the flow over a cylinder can produce a large region of separated
flow downstream of the cylinder (figure 10.4). This region is called a wake
(see also figure 8.3)..
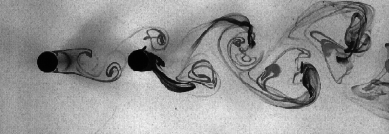 |
 |
Figure 10.4 Flow over two cylinders. From Japan Society of Mechanical Engineers.
|
The flow patterns over the front and back of the
cylinder are quite different. In the front, the flow smoothly passes over the cylinder,
but in the wake the flow is usually highly unsteady and large eddies
or vortices are shed downstream. The large eddies are formed at a regular frequency and
they produce pressure disturbances in the flow which we can sometimes hear as sound waves.
When we talk of the wind whistling in the trees, it is the sound of eddies being shed. A
Greek instrument, the Aolian harp, made use of this regular vortex shedding to produce
music. The alternate shedding of vortices produces an alternating lift force, oscillating
in direction and magnitude. If the frequency of the shedding couples to a natural frequency
of the cylinder and its supports, large cross-stream oscillations can occur in the cylinder
position. This kind of aerodynamic instability was responsible for the destruction of the
Tacoma Narrows bridge, and it has led to a number of spectacular cooling tower failures in
England.
Wakes almost always contain large eddying motions which are shed downstream, although
they may not shed at a regular frequency. For
instance, the wake of a boat often contains large eddying motions, and this region is often
called a ``dead-water'' region. Similar flow patterns are observed downstream of pylons
supporting a bridge.
In
any case, the eddies dissipate energy. Friction is the source
of the irreversibility of fluid flow: either through the formation of boundary layers over
the surface, or large eddies in the wake (in thermodynamics we say that the entropy of the
flow increases). In an imaginary fluid that has no friction (an inviscid fluid),
there would be no drag, the entropy does not change, and the flow would be reversible. For
example, a cylinder in the flow of an inviscid fluid has no vortex shedding and it has no
drag, contrary to our practical experience.
|
 |